V.S. Madhavan1, Hema Tresa Varghese2, C.Yohannan Panicker3* and Samuel Mathew4
1Deparatment of Physics, MES Ponnani College, Ponnani South, Malappuram, Kerala, India.
2Department of Physics, Fatima Mata National College, Kollam, Kerala, India.
3Department of Physics, TKM College of Arts and Science, Kollam, Kerala, India.
4Department of Physics, Mar Thoma College, Thiruvalla, Kerala, India.
Article Publishing History
Article Received on : 25 Jan 2013
Article Accepted on : 6 Mar 2013
Article Published :
Plagiarism Check: Yes
Article Metrics
ABSTRACT:
Salicylanilides possess a wide range of biological activities and they act as inhibitors of the two component regulatory system in bacteria. A natural bond orbital analysis has been performed in order to study intra-molecular bonding, interactions among bonds and delocalization of unpaired electrons for certain salicylanilide derivatives. Stability of the molecules arising from hyper conjugative interactions, charge delocalization has been analyzed. The electron density (ED) is transferred from the n(O), n(Cl) n(N) to the anti-bonding p*, s* orbital of the C-C ,C-O,C-N bonds. The results show that electron density in the anti-bonding orbitals and second order delocalization energies confirm the occurrence of intra-molecular charge transfer within the molecules.
KEYWORDS:
NBO analysis; Salicylanilides; DFT; Hyper-conjugation
Copy the following to cite this article:
Madhavan V .S, Varghese H. T, Panicker C. Y, Mathew S. Natural Bond Orbital (NBO) Analysis of Certain Salicylanilide Derivatives. Mat.Sci.Res.India;10(1)
|
Copy the following to cite this URL:
Madhavan V .S, Varghese H. T, Panicker C. Y, Mathew S. Natural Bond Orbital (NBO) Analysis of Certain Salicylanilide Derivatives. Mat.Sci.Res.India;10(1). Available from: http://www.materialsciencejournal.org/?p=295
|
Introduction
Natural Bond Orbitals (NBOs) are localized few-center orbitals that describe the Lewis-like molecular bonding pattern of electron pairs (or of individual electrons in the open-shell case) in optimally compact form. The natural bonding orbitals analysis has been performed in order to investigate intra-molecular charge transfer interactions, re-hybridization and delocalization of electron density within the molecules. In the NBO analysis1, 2 the electronic wavefunctions are interpreted in terms of a set of occupied Lewis type (bond or lone pair) and a set of unoccupied non- Lewis (anti-bond or Rydberg) localized NBO orbitals. The delocalization of electron density (ED) between these orbitals corresponds to a stabilizing donor acceptor interaction. Second order perturbation theory has been employed to evaluate the stabilization energies of all possible interactions between donor and acceptor orbitals in the NBO basis. The interaction result in a loss of occupancy from the localized NBO of the idealized Lewis structure into an empty non-Lewis orbital. The delocalization effects (or donor acceptor charge transfers) can be estimated from the off diagonal elements of the Fock matrix in the NBO basis. Salicylanilides (2-hydroxy-N-phenylbenzamides) show manifold pharmacological activities.3-8 The presence of amide (-NHCO-) groups in the structure of phenylcarbamoylphenyl-N-alkylcarbamates can cause interaction with various enzymes or enzymatic systems, for example, a carbamate moiety is characteristic for a number of herbicides acting as photosynthetic electron inhibitors.9,10 Imramovsky et al.,11 reported the photosynthesis inhibiting efficiency of a series of salicylanilide alkylcarbamates. Salicylanilides possess a wide range of biological activities and they act as inhibitors of the two component regulatory system in bacteria.12 Salicylanilides have been shown to be active against many pathogens, including Staphylococcus aureus,13 Escherichia coli, Pseudomonas aeruginosa,14 Aspergillus fumigatus,6 as well as being used as anthelminthics in veterinary practice.15 Salicylanilides have shown activity against M.tuberculosis16 although cytotoxicity in mammalian cells is a common problem. Salicylanilides have been suggested to target multiple specific targets in both prokaryotes and eukaryotes, including two component systems,7 transglycosylase,17 type III secretion systems,18 interleukin,19 and protein tyrosine kinase.20 In the present work, the NBO analysis of a series of Salicylanilides derivatives, 4-Chloro-2-(3- chlorophenyl carbamoyl)phenyl acetate, 4-Chloro- 2-(3,4 dichlorophenylcarbamoyl)phenyl acetate, 4- Chloro-2-(4-bromophenylcarbamoyl) phenyl acetate and 5-chloro-2-(3-chlorophenylcarbamoyl) phenylacetate are reported. Vibrational spectroscopic studies of these derivatives are reported by the authors previously.21-24
Computational details
The natural bond orbitals (NBO) calculations were performed using NBO 3.1 program as implemented in the Gaussian 09 package25, 26 at the DFT/B3LYP level in order to understand various second-order interactions between the filled orbitals of one subsystem and vacant orbitals of another subsystem, which is a measure of the intermolecular delocalization or hyper-conjugation. NBO analysis provides the most accurate possible ‘natural Lewis structure’ picture of ‘j’ because all orbital details are mathematically chosen to include the highest possible percentage of the electron density. A useful aspect of the NBO method is that it gives information about interactions of both filled and virtual orbital spaces that could enhance the analysis of intra and inter molecular interactions. The second-order Fock- matrix was carried out to evaluate the donor-acceptor interactions in the NBO basis.The interactions result in a loss of occupancy from the localized NBO of the idealized Lewis structure into an empty non- Lewis orbital. For each donor (i) and acceptor (j) the stabilization energy (E2) associated with the delocalization i ® j is determined as
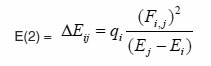
qi → donor orbital occupancy
Ei, Ej → diagonal elements
Fij → the off diagonal NBO Fock matrix element
In NBO analysis large E(2) value shows the intensive interaction between electron-donors and electron-acceptors and greater the extent of conjugation of the whole system. The second-order perturbation theory analysis of Fock matrix in NBO basis shows strong intra-molecular hyper- conjugative interactions of p electrons are given in Tables 1, 3, 5, and 7 and the NBO results showing the formation of Lewis and non-Lewis orbitals are given in Tables 2,4,6 and 8.
Results and Discussions
4-Chloro-2-(3-chlorophenyl carbamoyl)phenyl acetate
The intra-molecular hyper-conjugative interactions are formed by the orbital overlap between n(Cl) and π*(C–C) bond orbital which results in ICT causing stabilization of the system. The strong intra-molecular hyper-conjugative interaction of C5-C6 from of n3(Cl10) π*(C5-C6) which increases ED (0.3773e) that weakens the respective bonds leading to stabilization of 12.05 kcal mol-1. Also another intra-molecular hyper- conjugative interactions are formed by the orbital overlap between n(Cl) and π*(C–C) bond orbital which results in ICT causing stabilization of the system. The strong intra-molecular hyper- conjugative interaction of C18-C22 from of n3(Cl32) → π*(C18-C22) which increases ED (0.395e) that weakens the respective bonds leading to stabilization of 12.23 kcal mol-1. These interactions are observed as an increase in electron density (ED) in C-C anti-bonding orbitals that weakens the respective bonds. The strong intra-molecular hyper- conjugative interaction of C11-N13 from of n2(O12)→σ*(C11-N13) which increases ED (0.07808e) that weakens the respective bonds leading to stabilization of 25.38 kcalmol”.1 These interactions are observed as an increase in electron density (ED) in C-C anti-bonding orbitals that weakens the respective bonds. Again a strong intra-molecular hyper-conjugative interaction of O25-C26 from of n2(O31) → π* (O25-C26) which increases ED (0.13015e) that weakens the respective bonds leading to stabilization of 40.21 kcalmol-1. A strong intra-molecular hyper-conjugative interaction of C26– O31 from of n2(O25)→ π*(C26-O31) which increases ED (0.18625e) that weakens the respective bonds leading to stabilization of 38.14 kcal mol-1. Also a very strong intra-molecular hyper-conjugative interaction of C11-O12 from of n1(N13)→ π* (C11–O12) which increases ED (0.2505e) that weakens the respective bonds leading to stabilization of 39.49 kcalmol1.
The NBO analysis also describes the bonding in terms of the natural hybrid orbital n2(Cl10), which occupy a higher energy orbital (- 0.32380a.u.) with considerable p-character (99.98%) and low occupation number (1.97323a.u.) and the other n1(Cl10) occupy a lower energy orbital (-0.92204) with p-character (17.55%) and high occupation number (1.99332a.u.). Also n2(Cl32), which occupy a higher energy orbital (- 0.32450a.u.) with considerable p-character (99.98%) and low occupation number (1.97289a.u.) and the other n1(Cl32) occupy a lower energy orbital (-0.92336a.u.) with p-character (17.55%) and high occupation number (1.99329a.u.). Thus, a very close to pure p-type lone pair orbital participates in the electron donation to the σ*(C–C) orbital for n2(Cl10)→σ *(C–C) and σ*(C–C) orbital for n1(Cl32)→ σ *(C–C) interactions in the compound.
4-Chloro-2-(3,4-dichlorophenylcarbamoyl) phenyl acetate
The strong intra-molecular hyper- conjugative interaction of C4-C5 from of n3(Cl30)→π* (C4-C5) which increases ED (0.37305e) that weakens the respective bonds leading to stabilization of 10.16 kcal mol-1. Also another intra- molecular hyper-conjugative interactions are formed by the orbital overlap between n(Cl) and π*(C–C) bond orbital which results in ICT causing stabilization of the system. The strong intra-molecular hyper-conjugative interaction of C13-C17 from of n3(Cl31)→π*(C13-C17) which increases ED (0.45581e) that weakens the respective bonds leading to stabilization of 11.78 kcal mol-1. Another is from n(Cl) and π* (C–C) bond orbital which results in ICT causing stabilization of the system. The strong intra-molecular hyper-conjugative interaction of C13– C17 from of n3(Cl32)→π* (C13-C17) which increases ED (0.45581e) that weakens the respective bonds leading to stabilization of 11.74 kcal mol-1.
Again a strong intra-molecular hyper- conjugative interaction of C19-N21 from of n2(O20) →σ* (C19-N21) which increases ED (0.07320e) that weakens the respective bonds leading to stabilization of 22.25 kcal mol”1. A strong intra- molecular hyper-conjugative interaction of C24-O29 from of n2(O23) →σ*(C24-O29) which increases ED(0.20995e) that weakens the respective bonds leading to stabilization of 41.56 kcalmol-1. Also a very strong intra-molecular hyper-conjugative interaction of C19-O20 from of n1(N21)→σ*(C19–O20) which increases ED (0.25931e) that weakens the respective bonds leading to stabilization of 43.65 kcal mol-1. Again a strong intra-molecular hyper- conjugative interaction of O23-C24 from of n2(O29)→σ*(O23-C24) which increases ED (0.12495e) that weakens the respective bonds leading to stabilization of 38.71 kcal mol-1.
The NBO analysis also describes the bonding in terms of the natural hybrid orbital n2(Cl30), which occupy a higher energy orbital (- 0.32821a.u.) with considerable p-character (99.99%) and low occupation number (1.97714a.u.) and the other n1(Cl30) occupy a lower energy orbital (-0.94737) with p-character (14.27%) and high occupation number (1.99419a.u.).Also n2(Cl31), which occupy a higher energy orbital (- 0.33722a.u.) with considerable p-character (99.84%) and low occupation number (1.97504a.u.) and the other n1(Cl31) occupy a lower energy orbital (-0.95640a.u.) with p-character (14.30%) and high occupation number (1.99395a.u.). Also n2(Cl32), which occupy a higher energy orbital (-0.33356a.u.) with considerable p- character (99.82%) and low occupation number (1.97441a.u.) and the other n1(Cl32) occupy a lower energy orbital (-0.95127a.u.) with p-character (14.42%) and high occupation number (1.99395a.u.). Thus, a very close to pure p-type lone pair orbital participates in the electron donation to the σ*(C–C) orbital for n2(Cl30)→σ *(C–C) , σ* (C–C) orbital for n2(Cl31) →σ *(C–C) and σ*(C–C) orbital for n2(Cl32) →σ *(C–C) interactions in the compound.
4-Chloro-2-(4-bromophenylcarbamoyl)phenyl acetate
The strong intra-molecular hyper-conjugative interaction of C4-C5 from of n3(Cl20) →σ * (C4-C5) which increases ED (0.39490e) that weakens the respective bonds leading to stabilization of 10.08 kcal mol”1. Also another intra- molecular hyper-conjugative interactions are formed by the orbital overlap between n (O) and σ* (C–N) bond orbital which results in ICT causing stabilization of the system. The strong intra- molecular hyper-conjugative interaction of N22– C23 from of n2(O25) →σ*(N22-C23) which increases ED (0.07648 e) that weakens the respective bonds leading to stabilization of 26.06 kcal mol-1. These interactions are observed as an increase in electron density (ED) in C-C anti-bonding orbitals that weakens the respective bonds. Another intra- molecular hyper-conjugative interactions are formed by the orbital overlap between n(Br) and σ*(C–C) bond orbital which results in ICT causing stabilization of the system. The strong intra- molecular hyper-conjugative interaction of C13-C17 from of n3(Br21) →σ*(C13-C17) which increases ED (0.38778e) that weakens the respective bonds leading to stabilization of 9.09 kcal mol”1. Again a strong intra-molecular hyper-conjugative interaction of C27-O29 from of n2(O26)→π*(C27-O29) which increases ED (0.20971e) that weakens the respective bonds leading to stabilization of 43.54 kcal mol”1. A strong intra-molecular hyper- conjugative interaction of O26-C27 from of n2(O29)→π* (O26-C27) which increases ED (0.12474e) that weakens the respective bonds leading to stabilization of 41.46 kcal mol-1. Also a very strong intra-molecular hyper-conjugative interaction of C23-O25 from of n1(N22)→π*(C23-O25) which increases ED (0.28165e) that weakens the respective bonds leading to stabilization of 52.19 kcal mol-1.
The NBO analysis also describes the bonding in terms of the natural hybrid orbital n2(Cl20), which occupy a higher energy orbital (- 0.32870a.u.) with considerable p-character (100.0%) and low occupation number (1.97815a.u.) and the other n1(Cl20) occupy a lower energy orbital (-0.94563) with p-character (14.46%) and high occupation number (1.99428a.u.). Also n2(Br21), which occupy a higher energy orbital (- 0.29450a.u.) with considerable p-character (100.00%) and low occupation number (1.97873a.u.) and the other n1(Br21) occupy a lower energy orbital (-0.97568a.u.) with p-character (12.21%) and high occupation number (1.99530a.u.). Thus, a very close to pure p-type lone pair orbital participates in the electron donation to the→π*(C–C) orbital for n2(Cl20)→π* (C–C) and orbital for n2(Br21)→π*(C–C) interactions in the compound.
5-chloro-2-(3-chlorophenylcarbamoyl) phenylacetate
The strong intra-molecular hyper-conjugative interaction of C1-C6 from of n3(Cl10)→π *(C1-C6) which increases ED (0.3871e) that weakens the respective bonds leading to stabilization of 10.74 kcal mol-1. Also another intra-molecular hyperconjugative
interactions are formed by the orbital overlap between n(O) and π*(C–O) bond orbital which results in ICT causing stabilization of the system. The strong intra-molecular hyperconjugative interaction of C12-O13 from of n2(O11)→π*(C12-O13) which increases ED (0.213e) that weakens the respective bonds leading to stabilization of 45.25 kcal mol”1. These interactions are observed as an increase in electron density (ED) in C-C anti-bonding orbitals that weakens the respective bonds. Another intra-molecular hyperconjugative interactions are formed by the orbital overlap between n(Cl) and π*(C–C) bond orbital which results in ICT causing stabilization of the system. The strong intra-molecular hyperconjugative interaction of C23-C25 from of n3(Cl32)→π* (C23-C25) which increases ED (0.3906e) that weakens the respective bonds leading to stabilization of 10.53 kcal mol-1.Again a strong intramolecular hyper-conjugative interaction of O11-C12 from of n2(O13)→σ*(O11-C12) which increases ED (0.11944 e) that weakens the respective bonds leading to stabilization of 39.73 kcal mol-1. A strong intra-molecular hyper-conjugative interaction of C18-N20 from of n2(O19)→σ *(C18-N20) which increases ED (0.07389e) that weakens the respective bonds leading to stabilization of 23.51 kcal mol”1. Also a very strong intra-molecular hyperconjugative interaction of C18-O19 from of n1(N20)→π*(C18–O19) which increases ED (0.26075e) that weakens the respective bonds leading to stabilization of 47.65 kcal mol-1.
The NBO analysis also describes the bonding in terms of the natural hybrid orbital n2(Cl10), which occupy a higher energy orbital (-0.32467a.u.) with considerable p-character (99.98%) and low occupation number (1.97760a.u.) and the other n1(Cl10) occupy a lower The NBO analysis also describes the bonding in terms of the natural hybrid orbital n2(Cl10), which occupy a higher energy orbital (-0.32467a.u.) with considerable p-character (99.98%) and low occupation number (1.97760a.u.) and the other n1(Cl10) occupy a lower energy orbital (-0.94295) with p-character (14.73%) and high occupation number (1.99400a.u.). Also n2(Cl32), which occupy a higher energy orbital (-0.32921 a.u.) with considerable p-character (100.00%) and low occupation number (1.97808a.u.) and the other n1(Cl32) occupy a lower energy orbital (-0.94692a.u.) with p-character (14.68%) and high occupation number (1.99416a.u.). Thus, a very close to pure p-type lone pair orbital participates in the electron donation to
the σ*(C–C) orbital for n2(Cl10)→σ *(C–C) and σ*(C–C) orbital for n2(Cl32)→σ *(C–C) interactions in the
compound.
Conclusions
A natural bond orbital analysis has been performed in order to study intra molecular bonding, interactions among bonds and delocalization of unpaired electrons for certain salicylanilide derivatives. The electron density(ED) is transferred from the n(O), n(Cl) n(N) to the anti-bonding p*, s* orbital of the C–C ,C-O,C-N. Stability of the molecules arising from hyper-conjugative interactions and charge delocalization has been analyzed using natural bond orbital analysis. The results show that electron density in the anti-bonding orbitals and second order delocalization energies confirm the occurrence of intra-molecular charge transfer within the molecule.
References
- Reed,A.E.,Curtiss,L.A.,Weinhold,F.,Chem. Rev. 99: 899(1988).
CrossRef
- Chocholousova, J., Spirko, V.V., Hobza,P., Phys. Chem. Chem. Phys. 6: 22 (2004).
CrossRef
- Vinsova, J., Imramovsky, A., Ces. Slov. Farm.53: 294(2004).
- Vinsova, J., Imramovsky, A., Buchta, V., Ceckova,M.,Dolezal,M.,Staud,F.,Jampilek, J., Kaustova, J., Molecules 12:1(2007).
CrossRef
- Imramovsky, A., Vinsova, J., Ferriz, J.M., Dolezal,M.,Jampilek,J.,Kaustova,J.,Kunc, F., Bioorg. Med. Chem. 17: 3572(2009).
CrossRef
- A., Vinsova, J., Ferriz, J.M., Buchta,V.,Jampilek,J.,Bioorg.Med.Chem. Lett. 19: 348(2009).
- Ferriz, J.M., Vavrova, K., Kunc, F., Imramovsky, A., Stolarikova, J., Vavrikova, E.,Vinsova,J.,Bioorg.Med.Chem.18:1054 (2010).
CrossRef
- Otevrel, J., Mandelova, Z., Pesko, M., Guo, J., Kralova, K., Sersen, F., Vejsova, M., Kalinowski, D., Kovacevic, Z., Coffey, A., Csollei, J., Richardson, D.R., Jampilek, J., Molecules 15: 8122(2010).
CrossRef
- Abian,M.I.G.,Panzera,F.,Saez,J.L.,Abian, J.F.G., Torre, C., Martin, G.G., Protoplasma 204: 119(1998).
CrossRef
- Rao,V.S.,PrinciplesofWeedScience,ed.2, Science:Enfield,NewHampshie,UK(2000).
- Imramovsky, A., Pesko, M., Ferriz, J.M., Kralova, K., Vinsova, J., Jampilek, J., Bioorg.Med. Chem. Lett. 21: 4564 (2011).
CrossRef
-
El-Sayed, A.A., Dorgham, S.M., Kantouch, A., Int. J. Biol. Macromolecules 50: 273 (2012)
CrossRef
-
Macielag, M.J., Demers, J.P., Fraga-Spano, S.A., Hlasta, D.J., Johnson, S.G., Kanojia, R.M., Russell, R.K., Sui, Z., Weidner-Wells, M.A., Werblood, H., Foleno, B.D., Goldschmidt, R.M., Loeloff, M.J, Webb, G.C., Barrett, J.F., J. Med. Chem. 41: 2939 (1998).
CrossRef
-
De La Fuente, R., Sonawane, N.D., Arumainayagam, D., Verkman, A.S., Br. J. Pharmacol. 149: 551 (2006).
CrossRef
-
Chandrawathani, P., Yusoff, N., Wan, L.C., Ham, A., Waller, P.J., J. Vet. Res. Commun. 28: 479 (2004).
CrossRef
-
Waisser, K., Matyk, J., Divisova, H., Husakova, P., Kunes, J., Klimesova, V., Kaustova, J., Mollmann, U., Dahse, H.M., Miko, M., Arch. Pharm. (Weinheim) 339: 616 (2006).
CrossRef
-
Cheng, T.J., Wu, Y.T., Yang, S.T., Lo, K.H., Chen, S.K., Chen, Y.H., Huang, W.I., Yuan, C.H., Guo, C.W., Huang, L.Y., Chen, K.T., Shih, H.W., Cheng, Y.S., Cheng, W.C., Wong, C.H., Bioorg. Med. Chem. 18: 8512 (2010).
CrossRef
-
Dahlgren, M.K, Kauppi, A.M., Olsson, I.M., Linusson, A., Elofsson, M., J. Med. Chem. 50: 6177 (2007).
CrossRef
-
Brown, M.E., Fitzner, J.N., Stevens, T., Chin, W., Wright, C.D., Boyce, J.P., Bioorg. Med. Chem. 16: 8760 (2008).
CrossRef
-
Leichti, C., Sequin, U., Bold, G., Furet, P., Meyer, T., Traxler, P., Eur. J. Med. Chem. 39: 11 (2004).
CrossRef
-
Madhavan, V.S., Mary, Y.S., Varghese, H.T., Panicker, C.Y., Mathew, S., Van Alsenoy, C., Vinsova, J., Spectrochim. Acta 89: 308 (2012.
CrossRef
-
Madhavan, V.S., Varghese, H.T., Mathew, S., Vinsova, J., Panicker, C.Y., Spectrochim. Acta 72: 547 (2009).
CrossRef
-
Varghese, H.T., Panicker, C.Y., Madhavan,V.S., Mathew, S., Vinsova, J., Van Alsenoy, C., J. Raman Spectrosc. 40: 1211 (2009).
CrossRef
-
Panicker, C.Y., Varghese, H.T., Madhavan, V.S., Mathew, S., Vinsova, J., Van Alsenoy, C., Mary,Y.S., Mary,Y.S., J. Raman Spectrosc. 40: 2176 (2009).
CrossRef
-
Frisch, M.J., et al, Gaussian09, Revision B.01., Gaussian Inc, Wallingford CT (2010).
-
Glendening, E.D., Reed, A.E., Carpenter, J.E., Weinhold, F., NBO Version 3.1, Gaussian Inc., Pittsburgh, PA.

This work is licensed under a Creative Commons Attribution 4.0 International License.